Category:LowFrequency
Introduction, Low Frequency Observing (L, S & C Bands)
This document is intended for observers planning Karl G. Jansky Very Large Array (VLA) observations at low frequencies, specifically L (1-2 GHz), S (2-4 GHz), and C bands (4-8 GHz). These three receiver bands share at least some of the same problems and solutions, as compared to higher frequency bands. For an overview, general performance, and some specifics of receiver band (e.g. sensitivity, etc.) of the VLA, consult the Observational Status Summary.
Proposers should consider the overall observing strategy in detail at the proposal stage in order to accurately estimate the total time request and expected bandwidth after RFI has been taken into account. Below we begin with a general description of what to consider when proposing for low frequency (L, S, or C band) observations, followed by a more detailed discussion of the observation strategy for these bands, and an Observers Checklist.
Proposing for Low Frequency (L,S,C) Observations
In this section we will discuss several issues which should be considered when proposing for VLA low frequency (L,S,C) observations. All proposals should be prepared using the VLA Proposal Submission Tool (PST).
Observing Frequencies
All VLA antennas have been equipped with L and C band receivers. As of January 2012, 20 VLA antennas have S band receivers. The installation of the S-band receivers will be concluded by late Summer 2012. Plots of general receiver availability can be found here . Information on the sensitivity of the receivers over the frequency ranges can be found at the Observational status summary page.
Choice of Samplers: 8-bit vs. 3 bit, and Correlator Setup
The general capabilities for the 2013A semester (January 25 - April 22, 2013) are extensive with flexible tuning of sub-band spectral line windows using the 8-bit samplers (enabling up to 2 GHz total bandwidth), use of the 3-bit samplers at higher frequencies in a mode that is suitable for wide-band continuum and extragalactic lines and line searches, use of up to 3 independent sub-arrays and a phased array capability for VLBI.
Currently, the 3-bit sampler system is not as reliable as the 8-bit samplers. This is inherent in the sampler hardware. For the L (1-2 GHz) and S-band (2-4 GHz), which provide bandwidths of 1 and 2 GHz, respectively, the 8-bit samplers should be the obvious choice. For C-band (4-8 GHz) observations, the 3-bit samplers will provide a total frequency coverage of 4 GHz, while the 8-bit samplers will only deliver up to 2 GHz instantaneous bandwidth. However, with the current (less reliable) status of the 3-bit samplers, and the their requirement of increased overhead time for setup and calibration during the observations, will at best result in a marginal improvement in the RMS noise in spite of doubling the total bandwidth. Therefore, for all three receiver bands covered in this document, L, S, and C, we recommend the use of the 8-bit samplers.
For the correlator mode, you should pick the one that best fits your science case. For continuum science, choose the widest bandwidth per subband with coarse spectral resolution. For spectral-line, choose the subband bandwidth and the spectral resolution that better fit the scientific objectives of your project.
Issues that should be kept in mind are:
- The subbands in a baseband do not overlap. In addition, a few edge channels may need to be flagged because of the higher noise at the subband edges due to filter roll-off. Thus, if you have many spectral lines such that some of them lie near the edges of the subbands, consider tuning the 2nd baseband at a frequency that is offset by 1/2 a subband width with respect to the first baseband. This will give good noise performance across the width of a single baseband (see Figures 3 and 4 on the OSRO page).
- As described below, each subband must be calibrated independently. Thus if you chose a narrow subband-width for high spectral resolution, you must ensure that you pick flux density and complex gain calibrators that are strong enough for adequate S/N at this subband-bandwidth. See the Estimating Sensitivities section for more information on needed on-calibrator-times for successful calibration. Long slews will be needed to get to suitably strong calibrators.
- Note that it will most likely be necessary to Hanning smooth your data in order to get rid of Gibbs ringing (for the theory behind this phenomenon see, for example, http://en.wikipedia.org/wiki/Gibbs_phenomenon). Low frequency observations are prone to strong Radio Frequency Interference (RFI, see below), flagging the RFI will be close to impossible unless you first Hanning smooth your data. This needs to be taken into account when choosing the spectral resolution of your observations, since the effective resolution will be lower than the original, even though the number of channels will stay the same. Note that the frequency resolution (FWHM) of untapered spectra is (where is the channel spacing), while the resolution of Hanning tapered spectra is .
- Additionally, for spectral line observations, it is a good idea to pick a spectral resolution that will allow for at least 4-5 channels across your line, given its expected line width. Also here, very narrow and strong spectral features will suffer from 'Gibbs Ringing'. This is easily fixed by Hanning smoothing your data spectrally during data reduction, but you should account for the subsequent decrease in spectral resolution when picking your correlator configuration.
Radio Frequency Interference (RFI)
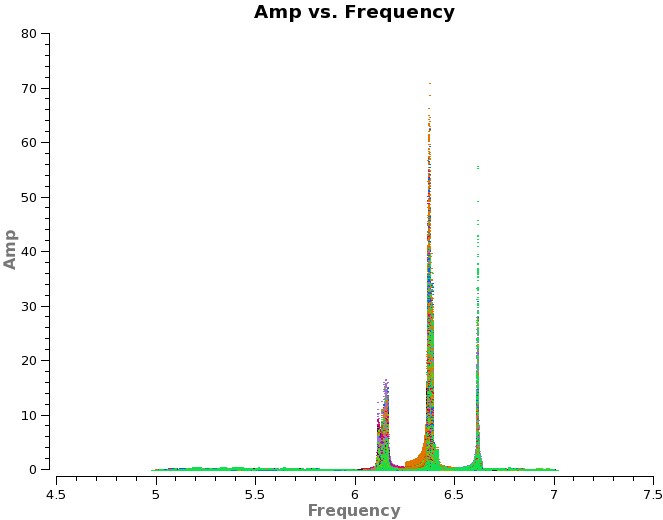
RFI is a major issue at L, S, and C bands, and should be accounted for properly. Of these three frequency bands, L band (1-2 GHz) is affected the most; up to 40% of this frequency band is contaminated by severe RFI. The affect of the RFI is worst in D-configuration observations. Extended array configurations are generally much less affected by RFI due to phase winding. Moreover, and in general, the RFI is worst at day-time when compared to night-time observations. Also, certain RFI is direction dependent.
Therefore, it is important to keep in mind that of the 1 GHz L-band bandwidth, ~600 MHz is the minimum useful bandwidth you will retain after flagging. The S-band (2-4 GHz) is also affected by severe RFI, and out of its 2 GHz bandwidth, ~1500 MHz is the minimum useful bandwidth you will retain after flagging.
Continuum observers wishing to use the full 1 GHz range of L-band, or the 2 GHz range of the S-band, should effectively use a narrower bandwidth in the sensitivity calculator (Estimating Sensitivities) while estimating the on-source time and the expected rms noise of their proposed observations, e.g., use 600 MHz and 1500 MHz instead of 1 GHz and 2 GHz, for L- and S-band, respectively.
As previously noted, RFI will also affect the spectral resolution of the data – in order to remove the Gibbs ringing of the RFI before flagging, the data will need to be Hanning smoothed. Please take this into account while planning and setting your observations.
Detailed information about the RFI at the VLA can be found here.
Configuration
The VLA is reconfigurable. In general, as the baseline length gets longer, the phase stability gets worse. The D-configuration provides the shortest baselines: poorest angular resolution, but highest surface brightness sensitivity. The A-configuration provides the longest baselines: highest angular resolution, but poorest surface brightness sensitivity. See the configuration schedule for details for each call for proposals.
It is generally important to consider
- What angular resolution is required for your science at the desired observing frequency
- For resolved sources, how does the desired angular resolution compare to the required surface brightness sensitivity -- particularly important for thermal emission
- How much flux will be resolved out by the array configuration that gives the desired angular resolution
- Will your source be up during a favorable time of day / month for your observing frequency?
The Observational status summary on resolution in conjunction with the Exposure Calculator, and the Monthly_Conditions_at_EVLA plots can help answer these questions.
Please note that low declination sources risk being subject to antenna shadowing at certain azimuths for the D configuration. These targets can still be observed, but the lower the declination, the smaller the windows of no-shadowing (one of either side of the north-south arm) will be, which effectively makes the setup of the scheduling blocks harder.
Estimating Sensitivities
The EVLA exposure calculator is an essential tool for estimating the required on-source observing time for the various parts of your observation. Also, see the RFI section above.
Below we describe important parameters to estimate various kinds of sensitivities.

All Sources
- Configuration: The configuration choice will not change the point-source rms noise, but it will affect the rms brightness temperature sensitivity which can be important for resolved sources and thermal emission.
- For the number of the available antennas at the L, S, and C-bands, check the EVLA Observational Status Summary.
- Elevation: System temperature increases at low elevations, especially at S-band. Due to ground spillover, S-band observations below 20 degree elevation is not recommended.
- Average Weather: This should not be a concern for low frequency (L,S,C band) observations.
- Be sure to pick the sky frequency (not the rest frequency) of your observations.
- Time on Source: If you are calculating rms based on a specified time, be sure to enter the appropriate time here. If you are estimating the noise in your science target field over the whole observation, use the total time on source for that field. However, if you are estimating sensitivities for self-calibration be sure to put what you expect the solution interval time to be. At minimum this would be the integration time.
Continuum Science Target:
- Enter the full continuum bandwidth per polarization decremented by the approximate bandwidth you expect for any line emission, and in particular RFI (see RFI section above)
- Enter dual polarization
Spectral Line Science Target:
- Enter the channel width you plan to use for imaging, i.e. can't be less than the channel width of the selected correlator mode, but could be larger if you plan to average channels during imaging.
- Enter dual polarization (unless you are using a single polarization correlator mode for additional spectral resolution)
Continuum Calibrator (Absolute Flux, Phase, Amplitude, Pointing):
- Select single polarization (each polarization product must be separately calibrated)
- Select subband width of your chosen correlator mode (each subband must be calibrated separately)
- For your calibration observations, it is generally a good idea to have a S/N ratio 4 on each baseline. You can use the exposure calculator to determine if the calibrator is appropriate for the selected bandwidth, e.g.:
- Put 2 in the Number of Antennas field
- Put the appropriate solution interval in the Time on Source field. This is typically the scan length at L, S, and C-band, but the specifics of your observations may require a different value.
- See if the S/N is 4 within the assumed solution interval.
Spectral Calibrator (Bandpass):
- Select single polarization (each polarization product must be separately calibrated)
- Select channel width of your chosen correlator mode (unless you plan to smooth before calibration; though other experimental options exist, typically you calibrate channel by channel)
- Again, it is generally a good idea to have a S/N ratio 4 on each baseline of your bandpass calibrator scans. You can use the exposure calculator to determine if the calibrator is appropriate for the selected bandwidth, e.g.:
- Put 2 in the Number of Antennas field
- Put the appropriate solution interval in the Time on Source field.
- See if the S/N is 4 within the assumed solution interval.
Low Frequency Observing Strategy
High solar activity results in emission that can severely affect the data to such a degree as to potentially render your observations useless. Also, such activity causes disturbing ionospheric effects. Therefore, it is imperative to avoid observing at L- and S-bands at day-time (including sunrise and sunset) during times of high solar activity. This is especially important now as we are nearing the max in the solar cycle in 2013. Solar flares with as much as a million Jy at L-band with narrow angular extents are a source of major interference. These flares are equivalent to bright unresolved sources with time-varying flux densities making it very difficult, if not impossible, to remove their effects. As a consequence, the resulting images will be of poor quality and low dynamic range.
Some links that can be useful are
- Solar activity monitoring: Solar activity and general space weather can be reviewed at the NOAA site. The site provides solar activity forecasts and geophysical (geomagnetic field) activity forecasts along with GOES X-ray flux values.
- Ionosphere monitoring: Global Ionospheric TEC maps.
The quiet Sun also poses problems for L-band observations, particularly in the short configurations (D and C). This would result in degraded image quality if the Sun is too close to the science target to within a few degrees. In the extended configurations (B and A), one can expect the effects of the quiet sun to be reduced.
Calibrations
A number of calibrator observations are needed for the subsequent data reductions. These usually include: Flux density calibrator, complex gain (phase and amplitude gain) calibrator, and polarization calibrator if polarization is part of your science objective. You will also need to observe a bandpass calibrator to correct for the delays as well as the relative gains of the spectral channels even if the observations are intended for continuum science. It is recommended that the flux calibrator be observed at least once in an observing run (a scheduling block). The flux calibrator itself, if desired, can be used to correct for the bandpass and antenna based delays, considering that the all the flux calibrators are strong sources at L, S, and C bands. Complex gain calibrators need to be observed before and after each target observation. The time required between phase calibrator observations depends on the array configuration (see below).
Absolute Flux Calibration
Accurate flux densities can be obtained by observing one of 3C286, 3C147, 3C48 or 3C138 during the observing run. Over the last several years, accurate source models have been implemented directly in AIPS and CASA for much improved calibration of the amplitude scales. Models of these calibrators at L, C, X, Ku, K, and Q bands, are available in both data reduction packages. Flux calibrator models for the S-band will be available in the near future. Meanwhile, observers can utilize the L-band or the C-band models of these calibrators for their S-band data.
In general, these calibrators are strong enough to be used for bandpass calibration as well. However, see the following section for the specifics on bandpass calibration, and whether your science goals require observing a stronger bandpass calibrator (i.e., if you are using very narrow channels). Note that bandpass calibration should be carried out even if the observations are intended for continuum science.
Bandpass Calibration
For the EVLA it is essential to calibrate the spectral response of your chosen correlator mode, even for continuum projects. However, the requirements for bandpass calibration are very dependent on your science goals/type of observation. Below we give some rules of thumb for different kinds of observations. If your project contains a number of aspects (spectral line and continuum), follow the recommendation that is the most limiting.
- Spectral Line Observations: Generally speaking, you want to obtain the same rms noise level per channel on your bandpass calibrator as you hope to achieve for your science target. In other words, your bandpass-calibrated science-target spectral-line data will be as noisy as the bandpass calibration applied to it (no matter how sensitive your science target observations originally were). Obtaining sufficient S/N for narrow bandwidth modes can be particularly challenging. For this reason you typically want to observe the strongest source available, preferably in the same part of the sky as your science target to minimize slew times.
- Caveat 1: If you know for certain that you will spectrally average your science target data, you can count on doing this for the bandpass calibrator too.
- Caveat 2: The bandpass response on the EVLA antennas are typically smooth over several MHz in bandwidth, suggesting that you can smooth the bandpass calibrator solutions spectrally a bit without averaging over instrumental spectral features that you want to calibrate out. This option is available in both AIPS and CASA.
- Continuum Observations: The requirements for bandpass calibration for (pure) continuum observations are significantly less stringent. A signal-to-noise per channel per antenna of about 20 is typically enough to ensure that each subband has adequate bandpass calibration. You may be able to go as low as a S/R of about 10 but this is a function of the science you want to do and should be carefully considered first.
Note that instead of using a single observation of a bright bandpass calibrator, it is also possible to use multiple observations (scans within a given scheduling block) of the complex gain calibrator to calibrate the bandpass by combining all its scans.
Complex Gain Calibration
In the choice of the complex gain calibrator, obviously a calibrator close to your target source will decrease slewing times. However, for the L, S, and C bands, we recommend choosing a strong P calibrator further away, rather than a weak nearby S calibrator. See the VLA calibrator manual for a description of the calibrator codes P and S.
The frequency (in time) of observing a complex gain calibrator in a given scheduling block depends on the configuration of the array. Rough guidelines for the typical time between complex gain calibrator observations are:
Array configuration | A | B | C | D |
---|---|---|---|---|
Time (min) | 10-15 | 20-25 | 30-40 | 50-60 |
Polarization Calibration
There are two components for polarization calibration:
- Determining the leakage terms (i.e., the polarization impurity between the R and L polarizations).
- Calibrating the absolute polarization angle.
There are two approaches to determine the leakage terms:
- either observe one or more strong calibrators (> 1 Jy) over a wide range (e.g., > 60 degrees) in parallactic angle and through multiple scans,
- or observe a strong unpolarized (typically less than 1% polarized) calibrator source through at least one scan.
To calibrate the absolute polarization angle, observe a calibrator with well-known polarization angle.
For detailed information on polarization calibration, including the most common calibrators, see the EVLA polarimetry guidelines.
Please note that avoiding day-time observations is even more critical for polarization observations, especially during peak solar activity, due to ionospheric Faraday rotation.
Correlator Integration Time
- The correlator integration time should be smaller than the time for significant phase variations, but ideally still yield a self-calibratable S/N on your weakest calibrator.
- The shorter the integration time the larger your dataset, so making the integration time unnecessarily short should be avoided.
- Amplitude loss due to time averaging (see Observational Status Summary, the section on time-averaging loss) should be taken into consideration while choosing the correlator integration time. For instance, 1 second in A-configuration, 3 seconds in B- and C-configurations, and 10 seconds in D-configuration, are recommended.
Overheads and how to split up observations
The calibrations described above will determine your overheads (except for slew time). However you also need to think about how you will chose to break up your observations. If you have many sources or need good uv-coverage it can be most convenient to observe in one long scheduling block (SB), or a few SBs if your source isn't up long enough for one track. This strategy also minimizes overhead. You can also choose to schedule an observation in smaller SBs, even if the source is up longer than the length of the SB. However, note that this will make the overhead larger.
The main point is that you need to plan ahead for this. Every scheduling block will need a complete set of calibrations as described above. Additionally, about 10 minutes is needed at the start of each SB for the setup of the system and moving to your first target. It is important to discuss these choices and the requisite overhead in your technical justification. For example, if your total time request including calibration time is 9 hours and you decide to break this up into 3 x 3-hour SBs, you will need to triple the original time estimate for the bandpass and flux calibrators since each need to be observed in each SB, plus add 30 minutes total for startup (i.e. 10 minutes each SB). Don't forget to take this additional time into account for the total time request at the proposal stage.
At these frequency bands, setup time, slew times, and observations of various calibrator sources (flux calibrator, bandpass calibrator, complex gain calibrators, etc...), will add at least 20% to 35% overhead, depending on the specifics of the observations and assuming scheduling blocks of more than 2 hours. If you have many objects at different locations to observe, these times could increased to up to almost 50%. Proposers requesting their observations to be carried out using shorter scheduling blocks should also account for higher overhead percentages.
Generally, do not scrimp on overheads, since they are important for adequate calibration. Note that strong calibrators require less on-source time than weak ones. Also, keep in mind that a decrease in time on source of the target will only correspond to a small increase in rms noise (the rms noise is inversely proportional to the square root of time). To achieve a more realistic estimate of the overhead, users can prepare scheduling blocks with various lengths at the time of writing the proposal, using the Observation Preparation Tool
OPT Observer's Checklist
Select calibrators
- Primary flux calibrators: Observe one of the four calibrators (3C286, 3C48, 3C138, or 3C147) to achieve absolute gain calibration.
- Complex gain calibrators: from the List of EVLA Calibrators, select compact calibrators that lie as close as possible to your target source (preferably less than 15 degrees for the L, S, and C bands).
- The smaller the source-calibrator angular separation, the better. However, in deciding between a nearby but weak 'S' calibrator, and a more distant but strong 'P' calibrator, the stronger 'P' calibrator is usually the better choice.
- Bandpass calibrators: Needed for both continuum and spectral line science. Could be the same as the flux calibrator.
Setup Instrument Configuration:
- For continuum science:
- Use available NRAO standard setups but consider time averaging; make sure you have short enough integration time in order to reduce time averaging losses.
- For Spectral line science:
- Velocity resolution: make sure the spectral resolution is enough to resolve your line (take subsequent Hanning smoothing into account).
- Make sure you have enough line-free bandwidth to measure the continuum flux.
- Correlator integration time: make sure you have short enough integration time in order to reduce time averaging losses, and to offset atmospheric/ionospheric phase winding.
Check your scheduling block (SB):
- General:
- Are your sources up? Check the Report of the SB.
- Are the scans sufficiently far away from zenith within the time range you observe? Even though this is not a major concern for L, S, and C band observations, we recommend not to observe within 5 degrees from the zenith.
- Are your source coordinates entered correctly and in the same epoch (J2000)?
- Have you integrated long enough to achieve your desired sensitivity on all sources (i.e., target(s) and calibrators)?
- Continuum considerations:
- Are your AC and BD IFs at different (non-overlapping) frequencies? See the EVLA Observations Status Summary for a description of the IFs AC and BD.
- Is your integration time short enough to reduce time averaging losses?
- Spectral line considerations:
- Are the rest frequencies of your lines correct?
- Is the velocity definition correctly specified?
- Is the rest frame correctly specified?
Post-processing guidelines
There are a number of useful tutorials to study in preparation for the data reductions. See for example:
- Wide-band wide-field L-band D-configuration imaging of supernova remnant G55.7_3.4
- Calibration and imaging of a single-pointing 6cm (C-band) EVLA wideband continuum dataset on the galaxy NGC2967, D-configuration
- Calibration and imaging of a C-band multiple-pointing EVLA continuum dataset on the supernova remnant 3C 391
This category currently contains no pages or media.